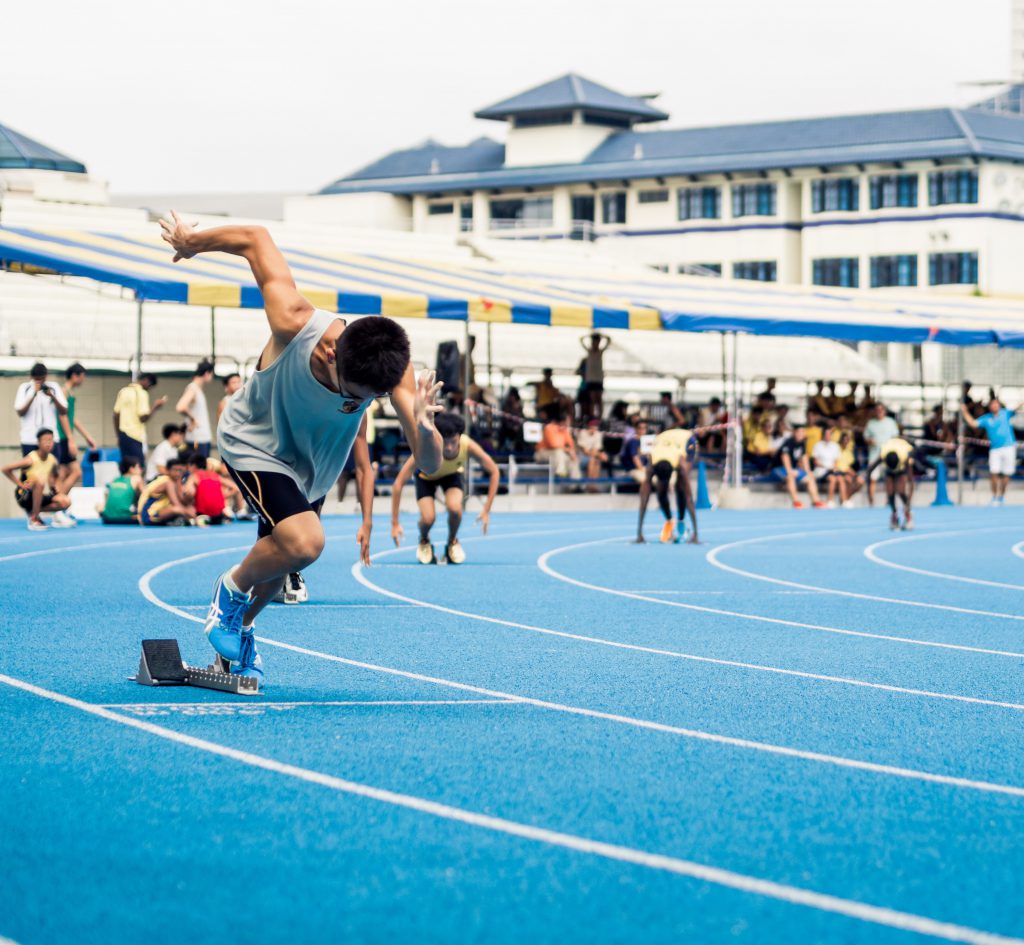
The hip joint is designed to withstand the stresses of human mobility. Joint damage due to excessive stress exceeding material thresholds leads to significant decreases in quality of life. In contrast, arthrosis-related joint damage eventually develops with ageing. The successful supply with a total hip endoprosthesis, therefore, strongly affects the mobility as well as the overall quality of life of patients. For good long-term results, stresses due to muscle and joint forces need to be defined during surgery planning. In this process biomechanical modeling can be of great value.
The supply of the hip with an endoprosthesis (total hip endoprosthesis) [HTEP]) is one of the most common orthopedic joint interventions [1,2]. Currently, there are more than 212,000 primary HTEP and more than 36,000 revision procedures performed in Germany annually [3]. The main positive outcomes of the HTEP supply consist of immediate pain relief and restoration of the functional joint mechanism. Thus, if the implant is positioned and aligned correctly, a good joint function is guaranteed [4,5]. However, through an endoprosthetic procedure the sensitive biomechanics of the natural joint mechanism can be severely impaired [5]. The goal of every procedure should therefore be the anatomical reconstruction of the hip joint, and with it, the muscular balance as close to the natural state as possible [1]. Therefore, the approximation of the physiological biomechanical relationships and dependencies (inclination and anteversion of the acetabulum, reconstruction of the hip rotation center, etc.) is of great importance for subsequent load stability and functionality of a HTEP [6]. Yet, the surgeon estimates the impact of an intervention on joint biomechanics mainly using personal experiences [2]. Neither does the surgeon have patient-specific information about the preoperative in vivo acting forces, nor does he know the changes in these forces due to the HTEP procedure. To solve this problem biomechanical modelling can be of great value. The domain of biomechanical modelling is the quantification and modeling of the preoperative in vivo existing forces and their potential changes due to the surgery. One main field of study is the development of modern methods and procedures for precise analysis and simulation of endoprosthetic fittings. Aspects of interest are, among others, movement optimization. („range of motion“ [ROM]), motion maximization or minimization of exposure. In general, the calculation of the numerical value and orientation of the resulting hip joint force R in relation to positioning and orientation of the implant is a major field of investigation.
In clinical practice, surgical planning of the implantation procedure is generally performed X-ray based. In addition to clinical parameters, important input data for the biomechanical models can be derived from the acquired X-rays. Regarding the modeling of the hip joint, numerous approaches have been published in literature. Important differences are X-ray image based (2D) modelling [7,8,9] and modelling based on 3D data (e.g. CT imaging). Furthermore, it needs to be differentiated between static and dynamic modeling [7,10,11]. The approaches significantly differ with regard to the necessary temporal and technical resources. This has a decisive influence on their applicability in the clinical routine. Various analyses of different modelling approaches for the calculation of the hip load, compared with corresponding in vivo data, have already shown a validity of the Pauwel modeling method [12,13]. Yet, the modelling approach by Pauwel, although relatively easy to use, is not – or only rarely – used in of today’s clinical practice [14].
The Pauwel model [16], which examines the mechanical causes of the femoral neck fracture and its healing has been further developed in recent decades with regard to its practical relevance in other areas [17]. The model (Fig. 1) assumes that the greatest strain on the hip joint during walking occurs in the stance leg phase (corresponding to the one-legged stand) [18,19]. This is made under the assumption that occurring inertia forces can be neglected for slow walking situations, therefore making the 2-D X-ray projection a reasonable approximation of the hip when standing on one leg [1, 3, 20]. Another assumption Pauwel introduced to simplify the calculation is that the change in direction of the muscle power FM_P of the combined abductors during the stance phase is relatively small and can therefore be neglected for the model [21]. The resulting hip joint force R is made up of the partial body weight force and the abductor muscle force. The partial body weight force can be calculated by subtracting the weight of one leg from the total body weight. In average, the remaining mass is 80% of the total body mass. R acts directly in the hip joint rotation center (HRC). The Pauwel model is predestined for a sensitivity analysis or a stress assessment, due to the high degree of abstraction and the therefore relatively simple technical implementation.
by medineer b
References
[1] Learmonth ID, Young C, Rorabeck C (2007) The operation of the century: total hip replacement. The Lancet 370(9597):1508–1519.https://doi.org/10.1016/S0140-6736(07)60457-7
[2] Leichtle U, Gosselke N, Wirth C, Rudert M (2007) Radiologische Evaluation der Variationsbreite der Pfannenpositionierung bei konventioneller Hüftendoprothesenversorgung. Fortschr Röntgenstr 179(1):46–52. https://doi.org/10.1055/s-2006- 927085
[3] Statistisches Bundesamt (2015) Gesundheit 2014. Fallpauschalenbezogene Krankenhausstatistik (DRG-Statistik) Operationen und Prozeduren der vollstationären Patientinnen und Patienten in Krankenhäusern. Ausführliche Darstellung. Fachserie12Reihe 6.4, Wiesbaden
[4] Thomas W, Schug M (1994) Über die Bedeutung der Position der Endoprothesenpfanne ausbiomechanischer und klinischer Sicht – Vorschlag einer Klassifizierung.BiomedTech(berl) 39(9):222–226
[5] Tschauner C, Hofmann S, Czerny C (1997) Hüftdysplasie. Morphologie, Biomechanik und therapeutische Prinzipien unter Berücksichtigung des Labrumacetabulare.Orthopade26(1):89–108
[6] Widmer K (2006) Die Hüfttotalendoprothese – Rekonstruktion der Biomechanik des Hüftgelenks. Articularis3:15–18
[7] Heller, M.O., Matziolis, G., König, C., Taylor, W.R., Hinterwimmer, S., Graichen, H., Hege, H.-C., Bergmann, G., Perka, C., Duda, G.N., 2007. Muskuloskelettale Biomechanik des Kniegelenks. Grundlagen für die präoperative Planung von Umstellung und Gelenkersatz. Der Orthopade 36, 628–634.
[8] Honl, M., Schwieger, K., Gauck, C.H., Lampe, F., Morlock, M.M., Wimmer, M.A., Hille, E., 2005. Pfannenposition und Orientierung im Vergleich. Navigation vs. konventionelle Handimplantion von Hüftendoprothesen. Der Orthopade 34, 1131–1136.
[9] Inaba, Y., Kobayashi, N., Ike, H., Kubota, S., Saito, T., 2016. The current status and future prospects of computer-assisted hip surgery. Journal of orthopaedic science : official journal of the Japanese Orthopaedic Association 21, 107–115.
[10] Kobayashi, N., Inaba, Y., Kubota, S., Higashihira, S., Choe, H., Ike, H., Kobayashi, D., Saito, T., 2018. Computer-Assisted Hip Arthroscopic Surgery for Femoroacetabular Impingement. Arthroscopy techniques 7, e397-e403.
[11] Affatato, S. (Ed.), 2014. Perspectives in total hip arthroplasty. Advances in biomaterials and their tribological interactions. Woodhead Publishing, Cambridge [England], Waltham, Massachusetts.
[12] Learmonth, I.D., Young, C., Rorabeck, C., 2007. The operation of the century. Total hip replacement. The Lancet 370, 1508–1519.
[13] Liu, Z., Gao, Y., Cai, L., 2015. Imageless navigation versus traditional method in total hip arthroplasty. A meta-analysis. International journal of surgery (London, England) 21, 122–127.
[14] Smith, A.J., Dieppe, P., Vernon, K., Porter, M., Blom, A.W., 2012. Failure rates of stemmed metal-on-metal hip replacements – Authors‘ reply. The Lancet 380, 106.
[15] Gross, T.P., Liu, F., 2014. Outcomes after revision of metal-on-metal hip resurfacing arthroplasty. The Journal of arthroplasty 29, 219–223.
[16] Affatato, S., 2014. The history of biomaterials used in total hip arthroplasty (THA). In: Affatato, S. (Ed.). Perspectives in total hip arthroplasty. Advances in biomaterials and their tribological interactions. Woodhead Publishing, Cambridge [England], Waltham, Massachusetts, pp. 19–36.
[17] Colic, K., Sedmak, A., Grbovic, A., Tatic, U., Sedmak, S., Djordjevic, B., 2016. Finite Element Modeling of Hip Implant Static Loading. Procedia Engineering 149, 257–262.
[18] Eschweiler, J., Hawlitzky, J., Quack, V., Tingart, M., Rath, B., 2017. Biomechanical model based evaluation of Total Hip Arthroplasty therapy outcome. Journal of orthopaedics 14, 582–588.
[19] Affatato, S., 2014. The history of total hip arthroplasty (THA). In: Affatato, S. (Ed.). Perspectives in total hip arthroplasty. Advances in biomaterials and their tribological interactions. Woodhead Publishing, Cambridge [England], Waltham, Massachusetts, pp. 3–18.
[20] Brinckmann, P., Frobin, W., Hierholzer, E., 1981. Stress on the articular surface of the hip joint in healthy adults and persons with idiopathic osteoarthrosis of the hip joint. Journal of biomechanics 14, 149–156.
[21] Buse, H., Feinle, P., 2016. Model System Studies of Wear Mechanisms of Hard Metal Tools when Cutting CFRP. Procedia Engineering 149, 24–32.